In the intricate world of electronic components, the stability of IGBTs (Insulated Gate Bipolar Transistors) stands as a cornerstone for system reliability. This article delves into the heart of a pivotal phenomenon within IGBTs: the latch-up effect. This effect rears its head when boundaries are crossed – specifically, when the safe operating area is exceeded, unleashing a torrent of runaway current. The roots of this occurrence intertwine not only with the chip's design but also with its intricate internal architecture. Despite the low odds of a latch-up failure in real-world scenarios, grasping its mechanics remains vital for the nuanced design and application of IGBTs.
Let's embark on a journey through the basic structure and operational principles of IGBT. A fusion of MOSFET and BJT traits, the IGBT emerges as a semiconductor powerhouse. Its blueprint mirrors that of a Darlington-connected Bi-MOS transistor. A forward voltage dance between the gate and emitter awakens the MOS transistor, paving a low-resistance path through the PNP transistor's base and collector. This act ignites the PNP transistor. The plot thickens as the gate voltage dwindles or reverses, extinguishing the MOS transistor and severing the PNP transistor's base current – thus, the IGBT dims. IGBTs, lauded for their voltage control prowess, are ubiquitous in power electronics.
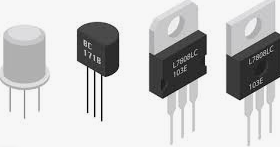
The latch-up effect, a tale of internal complexity, ties back to the IGBT's actual equivalent circuit. Hidden within are parasitic elements, such as covert thyristors. Excessive collector current breathes life into these components, triggering the NPN transistor. This event sets off a chain reaction, saturating both NPN and PNP transistors and awakening the parasitic thyristor into a self-sustaining lock – the latch-up. This scenario catapults the collector current, leading to a maelstrom of power consumption and, ultimately, device demise.
Beyond the static realm, the dynamic latch-up effect commands attention. During swift turn-offs, current's precipitous drop and high dv/dt spawn a displacement current. This interloper, coursing through the body region expansion resistance Rs, may stir the NPN transistor. Thus unfolds a dynamic self-locking saga, heightening the specter of device failure.
To counter the latch-up effect, a multipronged approach is key. Foremost, we must engineer IGBT structures resistant to this effect, perhaps by minimizing the body region extension resistance Rs. Subsequently, controlling the PNP transistor's hFE through the meticulous calibration of the n-buffer layer's thickness and doping level is crucial. Lastly, we can tame the PNP transistor's hFE with lifetime reduction techniques.
In conclusion, the safe working area is IGBT's Achilles' heel; straying beyond invites peril. From inception to final product, each step in the IGBT manufacturing odyssey is pivotal to harvesting top-notch components, tailored to diverse requirements. By unraveling the complex interplays within IGBTs, we unlock the potential to refine their design and usage, bolstering system dependability and efficiency.